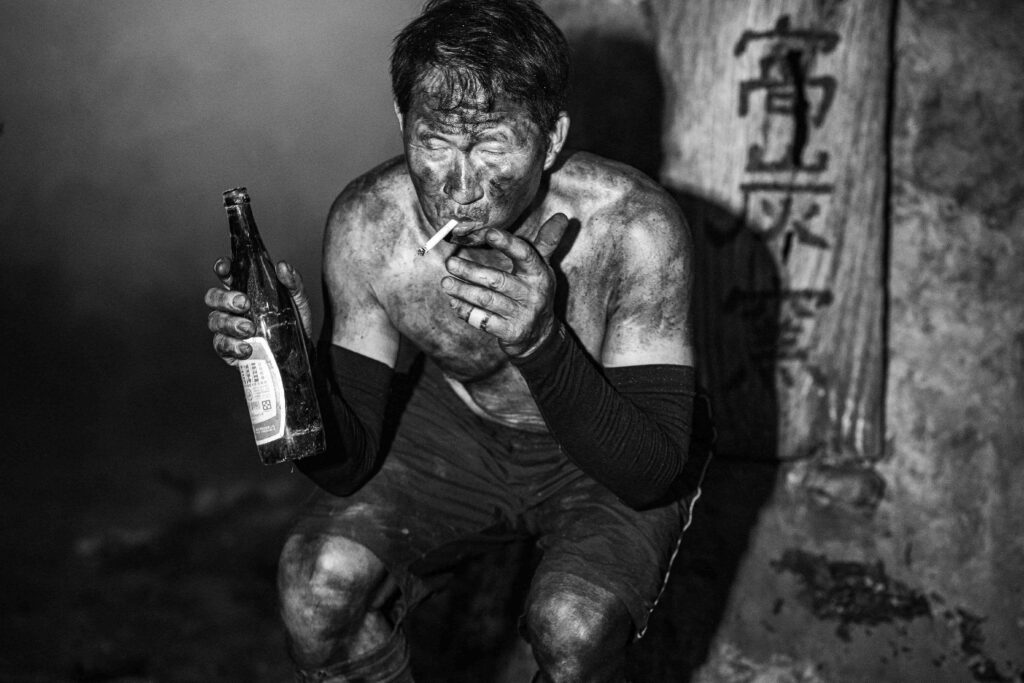
A May 16th article published in Science resounds our recently shared and deeply rooted thoughts on the need for innovation between government, academia, and industry to drive decarbonization.1 We recognize the substantial progress made in transitioning to clean energy sources like wind and solar. However, a full shift away from fossil fuels requires overcoming numerous obstacles. Writers from Science claim that two major challenges are finding alternatives for sectors that can’t be electrified and improving energy storage for intermittent renewables.2 A main driver in overcoming these challenges will likely be materials science innovation of the critical minerals driving the energy transition.
These critical minerals consist of base metals, energy transition metals, and rare earth elements–each with distinct roles in the sustainable resource transition. Base metals like copper, aluminum, and iron build renewable energy infrastructure and components such as wiring and weight-bearing structures.2 Energy transition metals like lithium, cobalt, nickel, and manganese, are critical for energy storage technologies like lithium-ion batteries used in EVs and grid-scale energy storage systems. 2 Rare earth elements (REEs), including neodymium, terbium, praseodymium, dysprosium, and the rest of the lanthanide series are vital for magnets used in wind turbines and EV motors. 2 In summary, base metals provide the structural and conductive components, energy transition metals enable energy storage, and rare earth elements enable efficient electric motors and generators.
Base metals are relatively accessible compared to energy transition metals and rare earth elements, which face an array of problems including scarcity, market dynamics, and ecological impacts. Additionally, these materials lack the established recycling systems of base metals like aluminum, increasing the reliance on extracting new materials. The exploration, mining, and processing of these materials can have severe environmental impacts like water pollution and soil contamination contributing to broader ecosystem disruption.3,4 Additionally, these minerals are often found in low concentrations, making extraction costly and resource intensive.5 To compensate for these costs; mining, extracting, and processing are often done in countries with regulations that are limited both environmentally and socially, leading to increased child labor and forced labor.6
Critical energy materials face scarcity from regional supply dominance, increasing demand, and market volatility. According to a 2024 U.S. Geological Survey Report, about 72% of US rare earths were imported from China in 2019-22, they mined roughly 69% of global extraction tonnage in 2022-3, and they also held roughly 40% of global rare earth reserves as of 2024.7,8 Not only dominating the extraction and ownership of critical minerals, China’s in-house share of refining is 50-70% for lithium and cobalt and almost 90% for rare earths.8,9 The only significant mineral processor beyond China is Europe, but they mainly process cobalt, mostly in Finland.9 As of 2022, Europe only processed 20% of global cobalt.9 Additionally, China’s relatively loose environmental and permitting regulations make it difficult for other regions to compete as the costs of ethical critical mineral sourcing are much higher.8
From November 2021 until now, lithium and other critical mineral prices have fluctuated significantly due to an oversupply situation caused by mining companies ramping up production too aggressively in response to high demand in 2021-2022.10 However, softening demand, especially for EVs led to a surplus and sharp price declines of over 75% for lithium by early 2024.10 Additional geopolitical tensions and regulatory uncertainties also contributed to the volatile pricing environment.10 In April of 2024, the US and UK placed sanctions on Russian aluminum, copper, and nickel, which has contributed further to the recent volatility of mineral markets. The price drops favor consumers, but they strain the long term prospect of investing in critical material exploration, therefore posing a future risk of mining and supply constraints.11
It’s a tough journey to secure and implement critical minerals as part of our climate and energy solutions. Increasing demand brings pressure to find, extract, and process more minerals, but China’s market dominance, broader geopolitics, regulations, and other struggles create highly volatile markets with considerable implications. Supplies are scarce and found in low concentrations, creating resource intensive operations budgeted at the cost of our people and ecosystems. The need for innovation is clear and we will discuss current and proposed solutions in a following writing.
REFERENCES
(1) Mirkin, C. A.; Sargent, E. H.; Schrag, D. P. Energy Transition Needs New Materials. Science 2024, 384 (6697), 713–713. https://doi.org/10.1126/science.adq3799
(2) Critical Materials Assessment; U.S. Department of Energy, 2023. https://www.energy.gov/sites/default/files/2023-07/doe-critical-material-assessment_07312023.pdf
(3) Talan, D.; Huang, Q. A Review of Environmental Aspect of Rare Earth Element Extraction Processes and Solution Purification Techniques. Miner. Eng. 2022, 179, 107430. https://doi.org/10.1016/j.mineng.2022.107430
(4) Not So “Green” Technology: The Complicated Legacy of Rare Earth Mining. Harvard International Review. https://hir.harvard.edu/not-so-green-technology-the-complicated-legacy-of-rare-earth-mining/
(5) The Not-So-Rare Earth Elements: A Question of Supply and Demand. Kleinman Center for Energy Policy. https://kleinmanenergy.upenn.edu/research/publications/the-not-so-rare-earth-elements-a-question-of-supply-and-demand/
(6) Carafano, J. Rare Earths Supply Chains and Confrontation With China. The Heritage Foundation. https://www.heritage.org/defense/commentary/rare-earths-supply-chains-and-confrontation-china
(7) Mineral Commodity Summaries: RARE EARTHS; U.S. Geological Survey, 2024. https://pubs.usgs.gov/periodicals/mcs2024/mcs2024-rare-earths.pdf.
(8) The Role of Critical Minerals in Clean Energy Transitions; International Energy Agency, 2021. https://iea.blob.core.windows.net/assets/ffd2a83b-8c30-4e9d-980a-52b6d9a86fdc/TheRoleofCriticalMineralsinCleanEnergyTransitions.pdf.
(9) Castillo, R.; Purdy, C. China’s Role in Supplying Critical Minerals for the Global Energy Transition; Brookings Institution, 2022. https://www.brookings.edu/wp-content/uploads/2022/08/LTRC_ChinaSupplyChain.pdf.
(10) Sharp declines in critical mineral prices mask risks of future supply strains as energy transitions advance – News. IEA. https://www.iea.org/news/sharp-declines-in-critical-mineral-prices-mask-risks-of-future-supply-strains-as-energy-transitions-advance
(11) Hoppe, J. Green Transition Set to Face Critical Minerals Shortfall, IEA Says. Wall Street Journal. May 17, 2024. https://www.wsj.com/articles/green-transition-set-to-face-critical-minerals-shortfall-iea-says-cc89acf0